Photobiomodulation (PBM) is a non-invasive, non-thermal form of light therapy that utilizes non-ionizing light sources to create therapeutic effects at the cellular level. Light sources employed in PBM include lasers and LEDs in the visible (400-700 nm) and infrared (700-1100 nm) spectra. PBM produces therapeutic treatments (PBMT) in diseased and damaged tissues to promote wound healing and tissue regeneration, reduce inflammation, relieve pain, and restore original cell function.
Figure 1 The Wide Range of Clinical Applications of PBMT
PBM was first observed by Endre Mester at the Semmelweis Medical University in Hungary when a faulty high-power laser, designed to destroy abnormal cells, instead emitted a low-power light. Mester observed how the low-level light promoted healing at an increased rate by stimulating skin cells and published his findings in 1967 (Gáspár, 2019). In 2015, PBM and PBMT(photobiomodulation therapy) became the recognized terms of choice in the field, standardizing the terminology for the therapy often referred to as low-level laser (or light) therapy (LLLT), cold laser therapy, or laser biostimulation (Anders et al., 2015).
In this article, we will delve into the fundamentals of photobiomodulation, exploring the mechanisms by which light interacts with cells and elucidating the underlying photochemical responses. We will shed light on the ongoing efforts to optimize PBM techniques and expand their therapeutic potential. Additionally, we will examine the current state of PBMT devices and what the future holds for light therapy.
An Introduction to Light Therapy
Photobiomodulation (PBM) is a non-invasive form of light therapy that utilizes non-ionizing light sources to create therapeutic effects at the cellular level. Light sources employed in PBM include lasers and LEDs in the visible (400-700 nm) and infrared (700-1100 nm) spectra. PBM produces therapeutic treatments (PBMT) in diseased and damaged tissues to promote wound healing and tissue regeneration, reduce inflammation, relieve pain, and restore original cell function.
PBM is classified as a non-thermal form of light therapy. Non-thermal light treatments do not produce significant heat during the procedure. Instead, they focus on utilizing other characteristics of light, such as wavelengths, to achieve desired therapeutic effects. While PBM doesn't intentionally produce heat (in contrast to photothermal modalities), heat generation remains possible in certain settings. If light intensity or exposure time is not appropriately controlled, excessive heat generation can occur. Heat generation is a pertinent obstacle for PBM, regardless of what wavelength is used.
The PBM process involves endogenous chromophores located in cells’ mitochondria resulting in photochemical changes. Photochemical changes are reactions or processes that occur as a result of the absorption of light energy and can lead to alterations at the molecular level (Anders, 2016).
After the light is absorbed, the light interacts with cytochrome c oxidase (Complex IV) in the mitochondria of cells to indirectly generate ATP (Energy-Carrying-Molecules), ROS (Reactive Oxygen Species), and NO (Nitric Oxide) via the electron transport chain. ATP, ROS, and NO impact all phases of wound healing (Lipko, 2021).
Light-Related Considerations
LED (Light-Emitting Diode) and laser (most often in the form of a laser diode) are the two primary light sources used for PBMT devices. While there is an overlap in application and attributes between LED and laser, there are also a few key differences.
Light Source
LEDs produce a relatively monochromatic light output either via selection of single color emitting semiconductor materials or by phosphor conversion. LEDs’ emission distributions are typically very diffuse and non-collimated.
Laser diodes are similar in nature to LEDs but contain additional resonant features, which ensure that the emission distribution is tighter than that of LEDs (in terms of wavelength, coherence, and emission angle). These features mean that the laser diode is generally more effective at penetrating deeper tissues to treat conditions under the skin.
Figure 2 Example Emission Patterns of Various Light Sources
Figure 3 Example Spectral Distribution, LED vs Laser
Cost
LEDs are inexpensive and ubiquitous, making them a great option for non-specialized clinics or personal devices, especially those focusing on surface-level treatments. Laser devices, especially those with higher power and specific wavelengths, tend to be more expensive.
LEDs are inexpensive and ubiquitous, making them a great option for PBM devices, especially those focusing on surface-level treatments.
Safety
There is a substantial and increasing body of literature that indicates that PBM is a safe and effective treatment option when following the recommended clinical parameters (Bensadoun et al., 2020). Individuals who intend to use PBM should discuss it with a medical professional.
Individuals who are photosensitive, have vascular or immunological disorders, silicone-reinforced tissue, or have undergone cosmetic surgery are at a higher risk for complications related to laser-based PBM (Khalkhal et al., 2020).
PBM devices that use Class 3 lasers (or higher) can cause eye damage if not handled correctly. Procedures for these devices should include instructions about how to prevent ocular damage (Edge-Hughes, 2020).
Both LED and Laser devices are considered to be generally safe for clinical and home use. PBM users can decrease their risk of PBM-related injuries by following the proper procedures and by working with a doctor.
Wavelength
LED devices typically offer a broader range of wavelengths, allowing for flexibility in targeting different depths and tissue types. Lasers, on the other hand, usually emit light within a narrow wavelength range.
Different wavelengths target distinct biological tissues and are important in determining penetration depth.
- Blue light (440 - 500 nm) can penetrate the dermal layers. Blue light has been shown to stimulate complex IV of the electron transport chain and also to activate endogenous porphyrins, which can be useful for antibacterial applications.
- Green light (500 - 570 nm) can penetrate the dermal and basal cells into the fat cells.
- Red light (620 - 750 nm), with the longest wavelength on the visible spectrum, can penetrate 0.5 to 1 mm deep into the fat cell layers. Red light is often chosen from the shorter end of this spectrum (~620nm-680nm) for stimulation of cytochrome c oxidase.
- Near-infrared light (750 - 3,000 nm) can penetrate the deepest and into the muscle and tissues 2mm before losing 37% intensity. NIR is often chosen from the shorter end of this spectrum (~760nm-820nm) for stimulation of cytochrome c oxidase. While NIR encompasses wavelengths up to 3,000 nm, wavelengths approaching and above 2,000 nm are absorbed by water in the skin resulting in dermal heating and potential burning sensations in the derma.
The different wavelengths of light and the depths they can penetrate are represented in Figure 4. This figure illustrates the tendency of skin pigments and blood to block the penetration of visible wavelengths. Infrared light is allowed relatively free passage; however, even it will be limited by absorption in water.
Figure 4 A Schematic of Penetration Depth in Each Wavelength
Where light is directed can create a variation in the effective absorption across the electromagnetic spectrum. Research shows transcranial light in the 700-750 nm wavelength tends to have little impact on brain structures, whereas 600-690 nm or 760-900 nm have a far greater impact. Research shows that using lightwaves at 830 nm allows 11.7% of light to penetrate the occipital region, 2.1% to penetrate the frontal region, and 0.9% to penetrate the temporal region (Hennessy 2016).
Power and Intensity
Laser devices generally have higher power output and intensity compared to LED devices. The concentrated and coherent nature of laser light allows for deeper tissue penetration and potentially more targeted treatment. LED devices, while less powerful, can still be effective for superficial treatments or larger treatment areas.
Readers may have noticed that power, intensity, wavelength, and light type all influence tissue penetration. These are just a few of the factors that influence PBM effectiveness at specific depths. Another factor that may influence penetration depth is the light delivery method used. Light delivery can be categorized as pulsed wave (PW) or continuous wave (CW).
Note: Further research to understand the optimal pulse parameters and their effects is ongoing.
Pulse wave PBM involves the delivery of light in a pulsed manner, where the light is turned on and off at specific intervals. Pulse parameters can include pulse duration (the length of time the light is on during each pulse), pulse frequency (the rate at which pulses are delivered per second), and duty cycle (the ratio of pulse duration to the total pulse period). Pulse PBM allows for precise control over the timing and duration of light exposure.
Constant wave PBM, also known as continuous wave PBM, involves the continuous delivery of light without any pulsation or interruption. In constant PBM, the light is emitted continuously throughout the treatment session.
While parameter standards regarding PW and CW are still being determined, it does appear that PW may be advantageous for improving light penetration into deeper tissues.
How Does Light-Tissue Interaction Affect PBM: Absorption, Reflection, and Scatter
PBM is typically used as a targeted treatment modality in which light needs to be focused primarily on the tissue of interest. Tissues are unique from person to person due to differences in blood content, water content, collagen content, and fiber development. In addition, the skin’s tone, condition, and thickness can contribute significant variability in optical response. Each application of PBM, from surface applications, like wound healing and pain management, to deeper targets, is affected by the properties of intervening tissues. Understanding how light and tissues interact can uncover obstacles.
Absorption, reflection, and scatter are three fundamental mechanisms by which light interacts with matter. It is important to consider these factors when applying light energy to living cells.
Absorption
Absorption occurs when light energy is absorbed by the cells or tissue it interacts with. When light is absorbed, it transfers its energy to the material. Absorption of photons allows the light source to create a therapeutic effect. Without absorption, there would be no energy transfer to the tissue, and the tissue would be unaffected by light. Ultimately, therapy is achieved when the target area absorbs light of the correct wavelength and intensity. However, absorption is a characteristic of all tissues, target or not, so a significant portion of the light waves are absorbed by incidental tissues and never actually make it to the target tissues (Anders 2016). Another unwanted side effect of unintended absorption occurs when the absorbed radiation inevitably converts to heat in the cells.
Reflection
Reflection occurs when light waves hit an object and bounce off its surface. The light’s reflected energy is not absorbed by the intended matter but is redirected. Reflective loss of therapeutic light is a particular problem at interfaces of tissue and air because the difference in refractive index is particularly large. As a result, variation can lead to a significant portion of the light being reflected away and wasted. Of course, these reflective losses will decrease the efficiency of the PBM device. PBM device designers need to consider which unintended targets might receive the reflected light, as this could interfere with sensors or, in extreme cases, impact eyesight.
Scattering
Scattering occurs when light waves interact with particles and are dispersed in different directions. Scattering happens upon total absorption and subsequent re-emission of light waves, whereas reflection occurs before absorption. The scattering of photons can provide feedback during therapy (Jacques et. al. 1987). Observing this process provides a diagnostic overview, as scattering depends on the tissue's ultrastructure and the presence of particles in the structure. Different wavelengths of light have different scatter properties when passing through living tissues (Jacques 1996).
Scattering is an ever-present hurdle. For example, A PBM device might generate a highly collimated beam, but this beam may begin scattering immediately upon entering the biological tissue, turning the tight beam into a rapidly diffusing cloud of photons. While this can be useful if the target is reasonably large and shallow, this effect is often an unwanted dilution of therapy.
Some devices use pulsed waves as a means to overcome biological barriers and increase therapeutic efficacy. Although there is no general consensus that pulsed is better than a continuous wave, limited evidence suggests this may be the case. Pulsed wave energy is typically less thermally stressful to the irradiated tissue and potentially improves deep tissue penetration.
Current State of PBM Devices
There are many PBMT devices on the market today. These devices are primarily for wound healing, pain management, and, most recently, for treating dementia and preventing mouth sores (Oral Mucositis) in cancer patients. Red light therapy devices, used for cosmetic purposes, are also available.
Highlighted below are companies that display innovation in the design and application of PBMT.
Thor Laser
Thor Photomedicine (aka Thor Laser) produces devices used in the treatment of pain in dental, oncology, and veterinarian settings. Their systems include the Thor Laser System, and different delivery tools are available to provide therapy by various means.
Figure 5 The Thor Laser System
Erchonia
Erchonia has PBMT devices that can be used to treat pain and a large variety of infections (i.e., bacterial, fungal, or viral). One of their products, the XLR8®, can be used to treat chronic/acute back, neck, and shoulder pain, as well as surgery-related pain. The device is handheld and battery-powered, allowing for treatment in hard-to-reach locations.
Figure 6 The XLR8® Laser
Aspen Laser
Apex Tri-Wave Laser Series by Aspen Laser features three therapeutic wavelengths that can treat pain and injuries. The wavelengths are 810nm, 980nm, and 1064nm. The Apex Laser Series focuses on providing safe and adjustable settings to allow for effective individualized treatments.
Figure 7 The Apex Tri-Wave Laser Series
Light Force Medical
Light Force Medical has several laser therapy systems available. Their LCT-1000, when launched, was the first Class IV medical therapy laser. It was granted FDA clearance in 2007 and delivers 10W of therapeutic power. The LCT-1000 comes with Light Force Medical’s patented laser-massage ball treatment head.
Figure 8 The LCT-1000 Therapy Laser
Vielight
Vielight has several devices for brain therapy. The Vielight Neuro Alpha 3 PBMT device is a pulsed device that emits light energy into the brain via the alpha frequency. Another device, the Neuro Duo 3, emits light energy at either alpha or gamma frequencies. Their devices work to enhance mental performance. Vielight has worked over the years to advance the brain space of PBM.
Figure 9 The Neuro Duo 3
Celluma
The Celluma PRO is a professional-grade LED light therapy device used for various skin treatments and pain management. It is a versatile device that employs three different wavelengths of light (465, 640, and 880 nm) to provide therapeutic benefits.
Figure 10 Celluma PRO
These devices, and others on the market, demonstrate how far innovation has come in the PBM field. PBM devices have evolved from large bulky systems designed for one treatment type to small handheld units and accessory ports that allow one system to be used for multiple treatment types. Companies leading the PBM field have many more devices aimed to help treat those with debilitating diseases going through clinical trials.
The Future of Photobiomodulation
With an increase in research being conducted, the future of PBM looks promising.
Perhaps the most exciting developments in this emerging field are the additional disease states which become open to treatment once optimal doses and delivery technologies are identified.
The most recent addition to the research landscape has been the research into PBM’s transcranial use in treating traumatic brain injury, major depressive disorder, dementia, Alzheimer’s, and more. Encouraging outcomes in animal studies has led to increased human studies.
Human studies investigating PBM for traumatic brain injury (TBI) show proper application can improve mental status. Naeser et al. (2011) showed significant cognitive improvement in TBI patients at a reduced treatment cost. A second study by the same group in 2014 showed improved learning ability that positively correlated with treatment duration. Other studies (Nawashiro et al., 2012; Henderson and Morries, 2015) reported decreased depression, anxiety, headache, and insomnia after PBMT. It is theorized that this reduction is a result of increased regional cerebral blood flow (Yang et. al. 2020).
Human studies into PBMT for Alzheimer’s disease, dementia, and major depressive disorder show various results. While animal studies show promise, Human studies are limited at this point, with small sample sizes. PBM’s effective use in animal trials encourages more human studies, hopefully with larger sample sizes and long-term follow-up.
Increased human studies allow researchers to understand better the parameters that influence therapeutic use and the obstacles that prevent effective therapeutic application. These parameters include wavelength, operation mode, power density, and treatment duration. The largest obstacle is when parameters must be tailored to an individual's biological structure to maximize effectiveness.
Given the considerable obstacles presented by the physics of biological tissue-light interaction, it could be tempting to think of the body as its own worst enemy when it comes to PBM therapy. However, as more and more becomes known about these interactions, designers are able to tweak their medical devices to make the most efficient use of the light they generate. As these factors become more understood and standardized, PBM’s ability to increase cerebral blood flow, enhance cellular metabolism, and prevent neurodegeneration in animal trials will transfer to human studies and, eventually, increased clinical use.
Innovation to overcome biological obstacles is evident in companies like Vielight, who have developed PBM technologies that use optimal transmission ‘windows’ for transcranial stimulation as well as pulsed wave technology for stimulating specific brain waves, or JustLight, whose Sunflower Rx device provides a convenient form factor for targeted PBM application as well as a custom combination of wavelengths for optimal light delivery (Serwer 2022). Additionally, Vielight is seizing on an even newer frontier in transcranial PBM therapy by integrating their device with cloud analytics of users’ EEG data, potentially closing the loop between dose and response. Vielight has already shown preliminary positive effects on Alzheimer’s patients, and JustLight is currently engaged in Alzheimer’s focused clinical trials (Vielight Research & Collaboration, n.d.).
Conclusion
In short, photobiomodulation (PBM) therapy is a promising non-invasive medical treatment that utilizes low-level light to stimulate cellular function and promote tissue repair. PBM is shown to have a variety of therapeutic effects, including reducing inflammation, accelerating wound healing, and improving muscle recovery. While more research is needed to understand its potential benefits fully, studies suggest that PBM may have applications for various medical conditions, including chronic pain, neurodegenerative diseases, and skin disorders.
However, there are still some challenges to overcome, such as a lack of standardized treatment protocols, difficulties in determining optimal application parameters, and understanding and identifying the variety in users' biological makeup and how it influences the effectiveness of PBM. With these challenges being addressed in research and device design, the future of PBM looks bright, and we will likely continue to see new applications and advancements in this field in the years to come.
References
Gáspár, L. (2019). Professor Endre Mester, the Father of Photobiomodulation. Journal of Laser Dentistry, 17(3), 146-148. laserpaintherapy. https://laserpaintherapy.com.au/wp-content/uploads/2017/10/Prof-Endre-Mester-Father-of-PBM.pdf
Anders, J. J., Lanzafame, R. J., & Arany, P. R. (2015). Low-level light/laser therapy versus photobiomodulation therapy. Photomedicine and laser surgery, 33(4), 183–184. https://doi.org/10.1089/pho.2015.9848
Anders, J. J. (2016, June 27). Photobiomodulation. American Society for Laser Medicine & Surgery, Inc. Retrieved April 4, 2023, from https://www.aslms.org/for-the-public/treatments-using-lasers-and-energy-based-devices/photobiomodulation
Nancy B. Lipko. Photobiomodulation: Evolution and Adaptation. Photobiomodulation, Photomedicine, and Laser Surgery.Apr 2022.213-233.http://doi.org/10.1089/photob.2021.0145
Bensadoun, R. J., Epstein, J. B., Nair, R. G., Barasch, A., Raber-Durlacher, J. E., Migliorati, C., Genot-Klastersky, M. T., Treister, N., Arany, P., Lodewijckx, J., Robijns, J., & World Association for Laser Therapy (WALT) (2020). Safety and efficacy of photobiomodulation therapy in oncology: A systematic review. Cancer medicine, 9(22), 8279–8300. https://doi.org/10.1002/cam4.3582
Khalkhal E, Razzaghi M, Rostami-Nejad M, Rezaei-Tavirani M, Heidari Beigvand H, Rezaei Tavirani M. Evaluation of Laser Effects on the Human Body After Laser Therapy. J Lasers Med Sci. 2020 Winter;11(1):91-97. doi: 10.15171/jlms.2020.15. Epub 2020 Jan 18. PMID: 32099633; PMCID: PMC7008747.
Edge-Hughes, L. (2020, July). Safety of Laser Therapy. https://physiotherapy.ca/. https://physiotherapy.ca/app/uploads/2022/07/safety_of_laser_therapy.pdf
Hennessy M, Hamblin MR. Photobiomodulation and the brain: a new paradigm. J Opt. 2017 Jan;19(1):013003. doi: 10.1088/2040-8986/19/1/013003. Epub 2016 Dec 14. PMID: 28580093; PMCID: PMC5448311.
Jacques S. Origins of tissue optical properties in the UVA, visible and NIR regions. In: Advances in Optical Imaging and Photon Migration. (Fujimoto J, Patterson MS, eds.) Optica Publishing Group, formerly OSA: Washington DC; 1996; pp. 364–371.
Naeser MA, Saltmarche A, Krengel MH, Hamblin MR, Knight JA. Improved cognitive function after, light-emitting diode treatments in chronic, traumatic brain injury: Two case reports. Photomed Laser Surg. 2011;29:351–358.
Nawashiro H, Wada K, Nakai K, Sato S. Focal increase in cerebral blood flow after treatment with near-infrared light to the forehead in a patient in a persistent vegetative state. Photomed Laser Surg. 2012;30:231–233.
Henderson TA, Morries LD. Multi-watt near-infrared phototherapy for the treatment of comorbid depression: an open-label single-arm study. Front Psychiatry. 2017;8:187.
Yang, M., Yang, Z., Wang, P., & Sun, Z. (2021). Current application and future directions of photobiomodulation in central nervous diseases. Neural regeneration research, 16(6), 1177–1185. https://doi.org/10.4103/1673-5374.300486
Serwer, J. (2022, June 30). JustLight pbc Developing Sunflower Rx™, a Breakthrough Medical Device Treatment for Alzheimer's disease. PR Newswire. https://www.prnewswire.com/news-releases/justlight-pbc-developing-sunflower-rx-a-breakthrough-medical-device-treatment-for-alzheimers-disease-301578854.html
Vielight Research & Collaboration (2023) Neuro RX Gamma - Pivotal Phase, National Library of Medicine. Available at: https://clinicaltrials.gov/study/NCT03484143 (Accessed: 01 July 2023).
Image References
Figure 1. Praveen, A. (2019, August 1). Phototherapy: Photobiomodulation therapy—Easy to do, but difficult to get right. Laser Focus World. Retrieved April 3, 2023, from https://www.laserfocusworld.com/lasers-sources/article/14037967/photobiomodulation-therapyeasy-to-do-but-difficult-to-get-right
Figure 4. The Impact of Near-infrared in Plastic Surgery - Scientific Figure on ResearchGate. Available from: https://www.researchgate.net/figure/A-Schematic-of-Penetration-Depth-in-Each-Wavelength_fig1_245539126 [accessed 3 Apr, 2023]
Figure 5. Image source: https://www.thorlaser.com/
Figure 6. Image source: https://www.erchonia.com/products/xlr8/
Figure 7. Image source: https://www.aspenlaser.com/apex-laser-system-series/
Figure 8. Image source: https://lightforcemedical.com/
Figure 9. Image source: https://www.vielight.com/devices/vielight-neuro-alpha/
Figure 10. Image source: https://www.celluma.com/products/celluma-pro
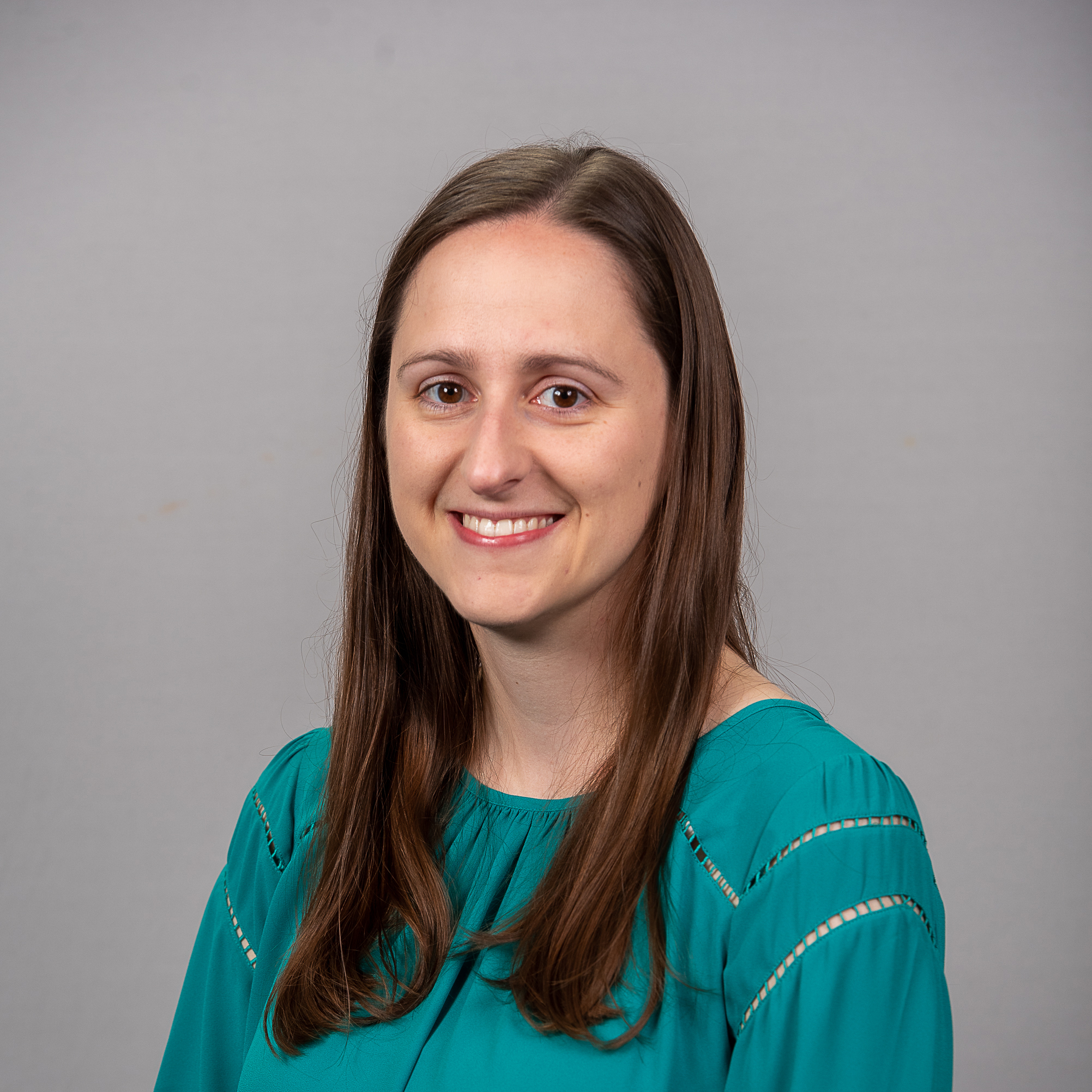
Kaity Peters
Kaity Peters is a design engineer at Lumitex. She works across Lumitex’s product lines developing products. Kaity uses her experience to develop medical devices from ideation to production release. Kaity has a Bachelor of Science in Biomedical Engineering from the University of Toledo.
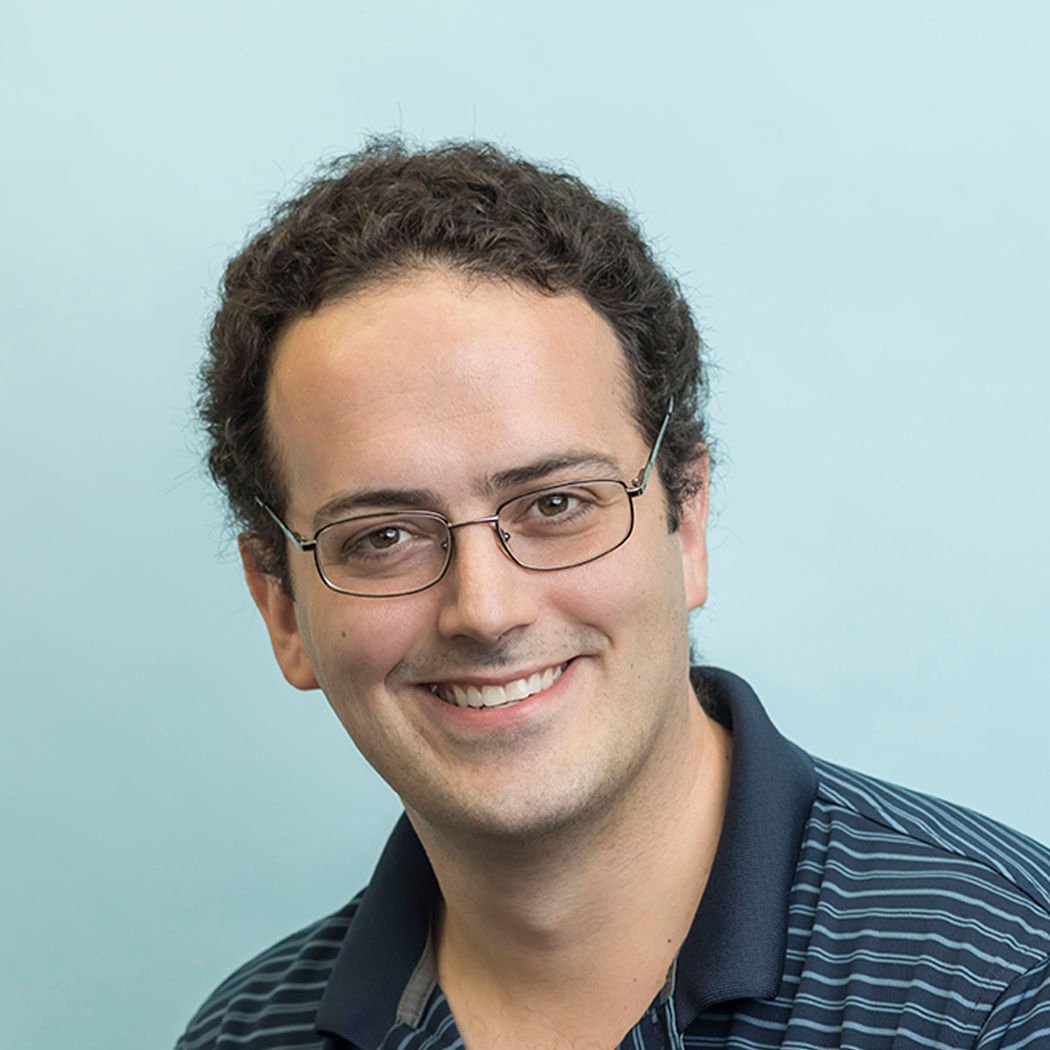
Mike Kerns
Mike Kerns is a Senior Design Engineer at Lumitex, where his first role was focused on the company’s line of legacy phototherapy products. Today, Mike is responsible for the research and development of new phototherapy applications and the general development of technology at Lumitex.
Comments